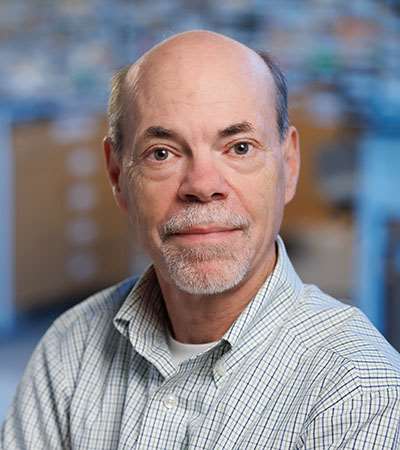
Mark T. McNally, PhD
Associate Professor
Locations
- Microbiology & Immunology
BSB B2235
Contact Information
General Interests
Education
Research Experience
- Alternative Splicing
- Avian Leukosis Virus
- Avian Sarcoma Viruses
- Cleavage Stimulation Factor
- Heterogeneous-Nuclear Ribonucleoprotein Group A-B
- Heterogeneous-Nuclear Ribonucleoprotein Group F-H
- Introns
- mRNA Cleavage and Polyadenylation Factors
- Polyadenylation
- Ribonucleoproteins, Small Nuclear
- RNA 3' Polyadenylation Signals
- RNA Precursors
Leadership Positions
- Chair, Course Evaluation Committee, Graduate School
- Course Director, IAHI, M1 Medical School
- Faculty Advisor, Research and Education Program, AHW
Research Interests
My laboratory uses molecular, genetic, biochemical, and cell biological approaches to study post-transcriptional mechanisms of gene regulation, including RNA splicing and polyadenylation control. One area of focus uses the simple retrovirus, Rous sarcoma virus (RSV), to understand the role of RNA processing in the virus life cycle. We are also exploiting antisense oligonucleotide technologies to alter RNA splicing as an approach to develop a breast cancer therapeutic.